Proof-of-Concept Study Advances Potential New Way to Deliver Gene Therapy
10/27/2022
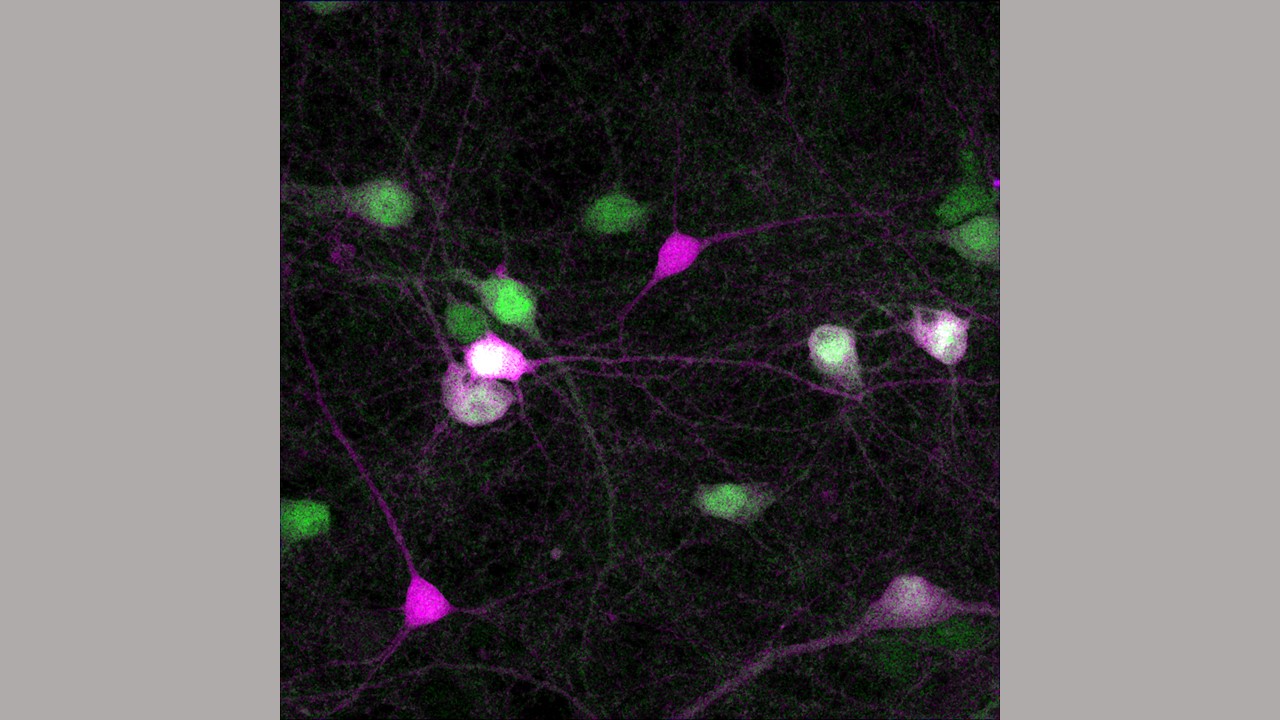
Johns Hopkins Medicine researchers say they have successfully used a cell’s natural process for making proteins to “slide” genetic instructions into a cell and produce critical proteins missing from those cells. If further studies verify their proof-of-concept results, the scientists may have a new method for targeting specific cell types for a variety of disorders that could be treated with gene therapies. Such disorders include neurodegenerative diseases that affect the brain, including Alzheimer’s disease, forms of blindness and some cancers.
For those looking to develop treatments for diseases where cells lack a specific protein, it’s critical to precisely target the cell causing the disease in each structure, such as the brain, to safely kickstart the protein-making process of certain genes, says Seth Blackshaw, Ph.D., professor of neuroscience in the Sol Snyder Department of Neuroscience and member of the Institute for Cell Engineering at the Johns Hopkins University School of Medicine. Therapies that don’t precisely target diseased cells can have unintended effects in other healthy cells, he adds.
Two methods currently used to deliver protein-making packages into cells vary widely in their effectiveness in both animal models and people. “We wanted to develop a gene expression delivery tool that’s broadly useful in both preclinical and clinical models,” says Blackshaw.
One current method of sending biochemical packages involves so-called “mini promoters” that direct the expression, or protein-making process of certain stretches of DNA. Blackshaw says this method often fails to express genes in the right cell type.
Another method, called serotype-mediated gene expression, involves delivering tools that latch on to proteins that stud the surface of certain types of cells. However, Blackshaw says such methods are hit-or-miss in their ability to specifically target only one type of cell, and they often fail to work in people even after successful testing in animal models.
The current proof-of-principle study, described Oct. 1 in Nature Communications, has roots in previous research by Johns Hopkins Assistant Professor of Pathology Jonathan Ling, Ph.D., who published “maps” depicting how various cell types use alternative splicing of messenger RNA, a cousin of DNA, to construct genetic templates that produce an ever-changing set of proteins in the cell. The changes depend on a cell’s type and location. Cells normally use alternative splicing to vary the types of proteins a cell can make.
Ling’s maps chart the patterns by which cells cut out introns, or extraneous sections of messenger RNA, and leave only the informative parts of genetic material, or exons, that actually express, or make, proteins.
However, introns are normally very large — sometimes millions of base pairs long and too big to package in currently available gene expression delivery systems. Ling found some 20% of alternative splicing patterns contained sections of intron DNA small enough to package into the gene expression delivery systems Blackshaw wanted to test.
Fortunately, for their purposes, the alternative splicing patterns were similar in both mouse and human DNA, and so potentially, applicable to both preclinical research and clinical use.
Together with then-postdoctoral fellow Alexei Bygrave, now an assistant professor at Tufts University, Blackshaw and Ling made packages of alternative spliced messenger RNA that could be delivered into cells via a benign virus. They dubbed the packages SLED, for splicing-linked expression design.
When the package slides into a cell, it opens there. Because the SLED system is not naturally integrated into the genome, the research team added genetic “promoters” that spark the production of proteins from the packaged SLED product.
The Johns Hopkins Medicine researchers constructed SLED systems for laboratory-cultured excitatory neurons and photoreceptors and were able to produce proteins exclusively in those cell types about half the time. Current minipromoter systems typically get the proteins in the right place about 5% of the time.
The team also injected SLED packages into mice with photoreceptors in the retina that lack a functional PRPH2 gene, which causes retinitis pigmentosa, a disease affecting the retina. The team found evidence that the SLED packages helped produce PRPH2 proteins in the photoreceptors of the treated mice.
In human ocular melanomas cultured in the laboratory, the scientists delivered SLED packages into only melanoma cells that lack the SF3B1 gene. The SLED package released RNA-producing protein that made the melanoma cells die.
Blackshaw says the SLED system’s best potential may be in combination with other gene delivery systems, and his lab is looking into methods to miniaturize introns to accommodate larger-size introns into SLED systems.
Blackshaw and Ling have filed for patents that involve SLED technology.
The research was funded by the National Institutes of Health (RF1MH123237, R24EY027283, K08EY027093, R01EY033103, 2T32EY007143), a Stein Innovation Award from Research to Prevent Blindness, the Wilmer Eye Institute, the National Science Foundation, a Johns Hopkins Kavli NDI Fellowship, and a Johns Hopkins IDIES Seed Fund.
Other researchers who contributed to the work include Clayton Santiago, Rogger Carmen-Orozco, Vickie Trinh, Minzhong Yu, Yini Li, Ying Liu, Kyra Bowden, Leighton Duncan, Jeong Han, Kamil Taneja, Rochinelle Dongmo, Travis Babola, Patrick Parker, Lizhi Jiang, Patrick Leavey, Jennifer Smith, Rachel Vistein, Megan Gimmen, Benjamin Dubner, Eric Helmenstine, Patric Teodorescu, Theodoros Karantanos, Gabriel Ghiaur, Patrick Kanold, Dwight Bergles, Ben Langmead, Shuying Sun, Kristina Nielsen, Neal Peachey, Mandeep Singh, W. Brian Dalton, Fatemeh Rajaii and Richard Huganir.