Researchers in infectious disease, oncology, and pulmonary are making some splashes in their study of epigenetics and what it portends for children’s health.
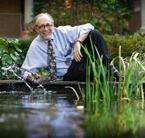
In clinic, pediatric pulmonologist Pamela Zeitlin sees two young boys who happen to have the same exact mutation in the gene responsible for cystic fibrosis, the inherited disease that causes severe mucous buildup in the lungs. Yet even a quick glance might reveal the two do not share the same severity of symptoms, for while one patient seems relatively healthy, the other clearly struggles to take a breath.
So, why do two young boys of similar age and background born with the identical genetic defect show such a disparity in their health? Why has the identity of the CFTR (cystic fibrosis transmembrane conductance regulator) gene as the underlying cause of the disease not been the cure-all that many researchers had hoped for? Rather than in the gene, the answer may lie quite literally above the gene, and involves an emerging field of science that pediatricians across Johns Hopkins are taking notice of. The answer could be found in epigenetics.
“Epigenetics is definitely the new frontier in understanding disease,” Zeitlin says. “The genes we have dictate how a disease manifests to some degree, and the environment does as well, but they don’t explain everything. I believe epigenetics will fill that gap.”
Biological Punctuation
What is epigenetics? Epigenetics (epi- is Greek for above) involves changes to our genes that do not involve changes to the sequence of DNA that makes up those genes. Rather, epigenetic changes involve a host of chemical modifications that serve to “mark” certain genes with a distinct signature. As Hopkins pediatric oncologist Robert Arceci explains it, “epigenetics is biological punctuation.” In writing, he notes, punctuation marks like commas, periods and apostrophes don’t change the actual letters in a piece of text, but they can significantly change the context. Take “doctors,” “doctor’s,” or “doctors’,” for example. The presence or absence of that single apostrophe changes how the word is perceived.
Epigenetic apostrophes serve a similar purpose. The number and location of these marks – which generally take the form of chemical groups with names like methyls, acetyls, and phosphates – control the extent to which that gene is turned on and makes its designated protein, much as a dimmer can adjust the intensity of a light bulb.
“These epigenetic signatures are important because without them all our cells would behave similarly,” Arceci says, “because every cell basically has exactly the same DNA.”
Through epigenetic changes, the genes in different cells – say heart cells and lung cells – can be fine-tuned to make them distinct. “So, having this level of regulation,” Arceci says, “makes all the difference between an embryo turning into a giant blob of cells versus a fully formed human that can walk, talk and think up equations like E=mc2.”
If this process goes awry, though, then cells can begin acting out of character, for example growing and dividing when they shouldn’t – the hallmark of cancer. In fact, it was through the study of this most complicated and insidious of diseases that epigenetics was born some 30 years ago.
People at the time knew that mutated and defective genes were important in triggering cancer, but the incredible heterogeneity of the disease – how similar cancers could spread and metastasize at different rates in different people, and why only some people responded to chemotherapy – hinted that there must be other factors that controlled its spread.
At Hopkins, two young scientists, Andy Feinberg and Bert Vogelstein, began exploring possible triggers for the rapid growth of cancer cells. In looking over normal and cancerous tissue samples, the pair discovered that in most cases the cancer samples had abnormally low levels of the aforementioned methyl groups around genes involved in regulating how cells divide. Feinberg and Vogelstein found that the DNA methylation was turning these genes off and keeping cell division in check; once removed, the cancer cells had free reign to proliferate like mad.
Since that early discovery the contribution of epigenetic changes to various types of cancer has become more and more pronounced, as Arceci found not too long ago in his studies of childhood leukemia. Childhood cancer is a maddening paradox; cancer is by its nature a disease of aging, built up over a lifetime of subtle changes and errors that occur each time a cell copies itself until a breaking point is reached. Yet in rare cases its strikes the young, and usually does so very aggressively.
“Unfortunately,” Arceci says somberly, “due to its comparative rarity, our understanding of childhood cancer is fraught with mysteries and inadequacies.”
A clue may have arrived in the form of PASG (Proliferation-associated SNF2-like Gene), a gene Arceci’s lab identified a few years back in looking at how leukemia cells reacted to survival factors called cytokines. That gene turned out to make a protein that was located in the nucleus, the heart of a cell where the chromosomes containing our genes are found. A closer study revealed that the PASG protein was involved in DNA methylation.
Arceci’s lab next decided to knock out, or eliminate, this gene in mice and see how they developed, and the researchers were surprised to see it induced premature aging. “Their litter-mates were nearby running and having a good time and these PASG-deficient mice were just growing old fast,” he says.
Intrigued, they looked over some more acute leukemia samples and found a high percentage of them had defective PASG, suggesting that this alteration could be involved in the etiology and/or outcome of such leukemias. Other investigators have linked senescence mechanisms to development and response to treatment of various cancers. Indeed, it appears that having high levels of the altered form of PASG in acute myeloid leukemia predicts an altered outcome and a possibly interesting approach to targeting leukemia by inducing senescence. Clinical trials are being planned.
But beyond an explanation, this discovery of PASG or other epigenetic factors in childhood cancers, such as those being studied by Arceci’s colleagues in pediatric oncology, Eric Schafer and Pat Brown, provides new hope for drug treatments. That’s because of one critical element of epigenetic defects that distinguishes them from genetic defects. Unlike DNA mutations such as those that contribute to cystic fibrosis, epigenetic changes are not inherently permanent. The various chemical groups that are used to mark genes are continually added and removed through an individual’s lifetime – from the womb to birth, adulthood and beyond.
Arceci notes an elegantly simple study conducted at Duke University in 2003 that highlights this malleable nature of epigenetics. The researchers fed female agouti mice – a strain predisposed to be yellow and fat – a diet rich in B vitamins like folate, and observed that the pups born of these mothers were browner and leaner than typical. Folate, among its many important roles, serves as a methyl source for gene methylation; these mice had a richer supply of methyls to regulate their genes, and in turn could tune down the agouti gene and maybe other genes contributing to their obesity.
Now, to infer that administrating a Bvitamin-rich diet could similarly help fight cancer is speculative at best, but Arceci notes the same principles of enhancing natural epigenetic properties to change gene function should apply: “The changes may not be as drastic as in the agouti mice, but maybe through a regimen of diet and drugs we could slow the progression of a leukemia or even induce remission.”
While his group is exploring possible drug candidates that enhance PASG activity, he’s more interested in delving deeper into what drives these epigenetic changes in high-risk pediatric cancers. Fortunately, he doesn’t have to do it alone. Culminating in the recent establishment of an epigenetics center headed by Feinberg, Hopkins has emerged as one of the preeminent world centers for studying epigenetic changes, with researchers across the medical school’s many departments working on identifying and manipulating these chemical marks.
“It may seem shocking, but we do talk to each other here all the time,” Arceci says. “And that high degree of interaction among such smart people will be critical if we are to define the key elements of these epigenetic changes. Only then can we not only better treat disease, but also anticipate disease, thus leading to prevention strategies.”
Isn’t that what pediatrics is all about, Arceci suggests. What specialty has been better at preemptive strikes against disease, be it with vaccinations or regular screenings?
“I always consider myself a failure when I see a child in the emergency room with cancer,” Arceci says “I think, I should have been able to do more to prevent that.”
Using CHARM to Map Epigenetic Changes
While the study with the agouti mice provides some clues into treating disease, it also reveals how small matters we might not think deeply about – like the amount of vitamins in our diet – could profoundly influence our epigenetic profiles. Diet, lifestyle, toxins, infections, all contribute to our epigenetic makeup. And that’s a big reason why pediatricians are, or should be, increasingly attuned to this field of research; for while the changes do occur over a whole lifetime, the periods of development, birth and childhood may be the most crucial in shaping who we are.
Pediatric infectious disease specialist Robert Yolken highlights his field of expertise, schizophrenia, as a prime example, usually when he’s asked why a pediatrician studies a disease that doesn’t manifest until 18 years of age or older and cannot be diagnosed in children.
“I always say schizophrenia may be an adult disease, but the seeds are sown during pregnancy or at childhood,” says Yolken. “Take winter births, preeclampsia and other birth complications, famine during pregnancy; all have been shown to have some association with increased schizophrenia risks, likely through epigenetic changes.”
Now, we might have to add early infection to that list. Yolken and his team recently made some headlines by finding a link between exposure to Toxoplasma gondii – a parasite that is transmitted through cats – and schizophrenia. The study showed that individuals infected with the parasite at an early age were twice as likely to develop schizophrenia. Initially, it may seem a bit crazy; bugs may make us feel sick, but can they change our personality and behavior?
This thinking is not without precedent, however. About a century ago about half the people locked up in insane asylums suffered from untreated syphilis, a sexually transmitted bacterial disease. And today, now that AIDS is becoming more manageable, physicians are finding an increased incidence of psychosis in HIV patients.
What really intrigues Yolken and colleague Sarven Sabunciyan, though, is figuring out how viruses or parasites can come in and alter behavior. And the evidence points to epigenetic changes.
“Think about what a virus’ goal is when it infects a cell,” neurovirologist Sabunciyan explains. “It wants to hijack the cellular machinery to turn on genes that can help it replicate itself and infect more cells. And we know from a lot of cancer and development studies that targeting the epigenetic patterns is a principal way to reprogram a cell.”
To delve into this, Yolken and Sabunciyan were part of a team that developed Comprehensive High-Throughput Arrays for Relative Methylation, or CHARM. It’s a high-tech technique that enables them to scan millions of bits of DNA sequence to map out the sites of methylation in an entire genome.
With CHARM, they’re taking a two-pronged strategy to map epigenetic changes associated with schizophrenia. One, they infect healthy brain cells in laboratory dishes with Toxoplasma to characterize what changes in methylation the parasite can induce. At the same time they’re mapping out post-mortem brain samples of schizophrenics, to see how their methylation patterns are altered compared to healthy individuals. Then, the different maps can be placed side-by-side, so to speak, to see which changes in schizophrenia might have been a result of infection.
Eventually, this could lead to a host of informative maps linking various infectious agents to risks for mental disorders. Thus, when a child comes in with an infection, doctors can plan ahead to prevent any future diseases from manifesting. Sabunciyan tempers this optimism by noting it will take some time to generate a clear map.
“We have no idea how complex these epigenetic changes are. If Toxoplasma affects everyone the same, I could probably run 10 samples and get an idea of what the changes are,” he says. “But mental diseases like schizophrenia are very heterogeneous and the underlying epigenetics may be only subtly different, so it likely will involve hundreds of samples or more.”
And though an ingenious approach, using CHARM to make deductive inferences about how epigenetic changes occurred isn’t the most ideal method to identify links with infection. “What we really need are prospective studies, where we can enroll kids and follow them over time, to truly understand how genetics and environment can alter epigenetic profiles,” Yolken says.
Obviously, because epigenetics is a new field of science such trials haven’t been available, but with the initiation of the National Children’s Study (NCS) last year, that will change. The largest and most comprehensive effort ever to link environment, lifestyle, and genetics to overall health, this National Institutes of Health study plans to follow 100,000 babies in over 100 countries from birth to age 21 years. At periodic intervals, researchers will collect biological specimens from the babies and both parents, as well as take environmental samples from around the house. Family dynamics, community and cultural influences will also be examined. Future scientists, therefore, should have a treasure trove of information to help them unlock the epigenetic code, and Yolken thinks that the information he and Sabunciyan obtain will be valuable in helping those scientists work it out.
“There was hope that sequencing the human genome would answer a lot of questions, and while it clearly didn’t tell the entire story, it was valuable in helping us in our work,” Yolken says. “Likewise, while our studies may not have that immediate impact in clinics, they will be instrumental down the road. Together with the genetics we already have and the NCS, the future in treating or preventing schizophrenia and other mental disorders is promising.”
The New Frontier
As for Pamela Zeitlin, the future looks hopeful as well. While scientists have spent most of the past 20 years chasing the genetics of CF to try and develop successful gene therapies, epigenetics is taking hold as the new frontier of pulmonary disorders.
It makes sense in a way, for while epigenetics have a hand in most any disorder, the cells in our lungs and nasal passages are constantly exposed to environmental stresses and epigenetic modifiers like toxins or infectious agents. For example, recent work is now confirming that cigarette smoke induces dramatic epigenetic changes in the lungs in addition to its potential for causing genetic mutations. By that same token, spray inhalers with chemicals that induce beneficial epigenetic changes could be used to improve the health of CF patients, or patients with other pulmonary conditions like asthma.
“From a delivery standpoint it would be much simpler than gene therapy,” Zeitlin says, “which is exceptionally tough because you have to periodically get a good copy of your desired gene into inflamed lung cells.”
Zeitlin entered the field quite serendipitously several years ago. Other scientists had been trying to express healthy CFTR genes in diseased cells by attaching the genes to small pieces of viral DNA, or vectors, and turning the gene on with a chemical called 4-phenylbutyrate (4-PB).
But Zeitlin noticed that even in cells that did not have any gene vectors, the butyrate was still correcting the defects a bit. Curious, she looked closer and found that butyrate was inhibiting a protein called a histone deacetylase, or HDAC. This protein is a bit like Arceci’s PASG, but instead of adding methyl groups to genes, HDACs take another chemical group called acetyls off of the genes. The end result is similar, though, with gene activity being changed, in this case to enable cells to turn on genes that can compensate for defective CFTR.
Why is this important? Some early clinical trials with oral 4-PB showed that it could improve nasal function in CF patients, though it did require large doses and a by-product of the drug produced an unpleasant odor. Still, over the years Zeitlin has been working on tweaking the 4-PB molecule to create next-generation drugs that have better efficacy and fewer side effects. She hopes to start more trials soon.
Like Arceci, Yolken and Sabunciyan, Zeitlin is being proactive, working with researchers in geneticist/pediatrician Gary Cutting’s lab to scan the blood of twins and siblings with CF for possible epigenetic patterns that might correlate with disease severity. Indeed, they are all taking on quite ambitious projects. But these dedicated physician scientists wouldn’t want it any other way, notes Arceci, recalling a memorable anecdote from his younger research days at the Marine Biological Laboratory in Woods Hole, Mass.
“Often in walking to campus I would see Albert Szent-Györgyi, a great scientist who had won the Nobel Prize years earlier for discovering vitamin C, fishing in this fairly small pond. And he always used a big lure and, not surprisingly, would always come away empty handed. He was always asked why he kept fishing like that when there likely weren’t any fish big enough to eat his lure in that small pond. He always replied, ‘It’s always more fun to not catch a big fish than to catch a little fish.’
“Epigenetics,” Arceci says, “is that big fish. And we have to dream big to catch it.”
Science writer Nick Zagorski, a regular contributor to Johns Hopkins publications, can be reached at [email protected]