The Story of Epigenetics
The Software in Cancer Cells

JOHN HERSEY C/O THEiSPOT
Epigenetics is the software package. Researchers believe that every cancer may have 50 to several hundred genes that have working “hard drives,” but their epigenetic “software” is causing them to act in a way that can lead to cancer development.
Renowned veteran cancer scientists such as Donald Coffey, Stephen Baylin, Peter Jones, Andrew Feinberg and current Kimmel Cancer Center Director William Nelson have been studying this biological process for decades.

As a field of study, epigenetics did not gain widespread acceptance until the early 2000s. Technologies that allowed science to analyze DNA at the molecular level and the tenacity of a relatively small group of scientists proved its validity. The long-concealed mysteries of what some have referred to as the “ghost in our genes,” referring to epigenetic mechanisms’ ability to alter gene expression without leaving a permanent mark on DNA, were uncovered.
With a group of epigenetic scientists, whom Nelson characterized as “second to none,” the Kimmel Cancer Center became a hub for epigenetic discovery and clinical translation.
Findings by Feinberg, director of the Johns Hopkins Center for Epigenetics in the Institute for Basic Biomedical Sciences, and Baylin, the Virginia and D.K. Ludwig Professor of Oncology, blazed trails in this field.
Feinberg described a global demethylation of the cancer genome. In normal human development, when the sperm and egg come together and form that first cell, how that one cell divides and determines what its fate will be to eventually form a complete human body was controlled through epigenetic mechanisms, he showed. Gene expression is what makes a cell behave the way it behaves, but how a cell figures out what proteins to express is controlled through epigenetics.
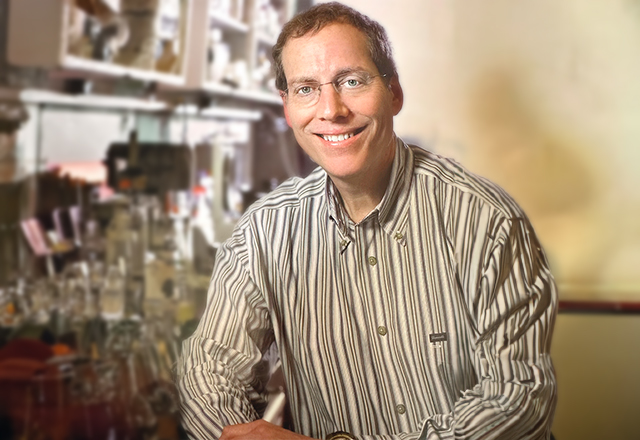
Feinberg suspected that this process was somehow getting hijacked in cancer. Corruption of the mechanisms that makes an undifferentiated cell know to become a liver cell could be at the root of the transformation of that same liver cell into a cancer cell.
Baylin’s focus was on chemical changes to protein-expressing regions of cancer genes, mainly tumor suppressor genes. The chemicals act like punctuation marks, turning off or accelerating gene expression, and provided a therapeutic target. Drugs that blocked methylation of the gene could, in principle, turn a tumor suppressor gene back on or a tumor accelerating oncogene off.
These promising advances inspired the research of young investigators entering the cancer field.
Kimmel Cancer Center Director William Nelson, M.D., Ph.D., was one of them. He did not set out to become an epigenetics researcher. In the early 1990s, he was beginning his career as a prostate cancer clinician and scientist when his research on cancer drug resistance led him to what remains today as one of the most classic examples of gene silencing through hypermethylation driving the development of cancer.
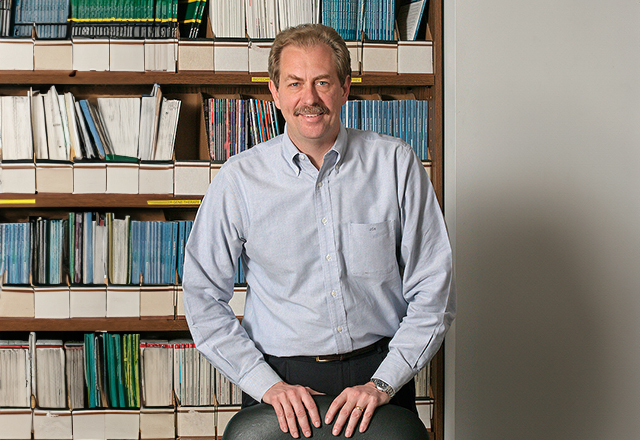
Baylin and former Kimmel Cancer Center faculty member James Herman, M.D., had already introduced a scenario in which tumor suppressor genes could be rendered inactive through the epigenetic process of a chemical change to DNA, called hypermethylation, but they had not uncovered a real-life example.
Nelson’s research led him to a gene called GSTP1, which he found was hypermethylated in prostate cancer. His discovery was used to create the first noninvasive, epigenetic-based test for the disease.
Baylin and Herman built a tool that allowed scientists to look laterally at many genes across many cancers and establish a pattern of silencing through gene methylation. These hypermethylated genes were the subjects of promising innovation in the form of biomarker tests that could tease out aggressive cancers from more indolent forms and provided new targets for novel treatment strategies.
Laboratory findings in leukemia and lung cancer paved the way for clinical trials of a drug that appeared to have the ability to fix some of the epigenetic-initiated changes to genetic code that helped cancers grow and thrive.
The world was beginning to take notice, and Baylin’s laboratory model was becoming a clinical model. Crucial to these advances was a new type of drug recognized by Jones in the 1970s as a demethylating agent. Too much methylation in the active regions of tumor suppressor genes was found to shut the genes down, giving advantage to one of the cancer cell’s iconic behaviors — uncontrolled growth. Blocking the methylation of the gene turned the suppressor gene back on.
Research published in the 1980s led Baylin and team to take a closer look at a demethylating drug called 5-azacytidine, which had largely been abandoned because of its toxicity. Laboratory studies in lung cancer and leukemia led to a clinical trial for patients with a pre-leukemia condition called myelodysplastic syndrome (MDS). The drug worked well, with some patients disease-free for 10 years and counting. Based on this work and the research of others, including former Kimmel Cancer Center faculty member Jean-Pierre Issa, 5-azacytidine received FDA approval for treatment of MDS. Baylin wondered if the drug might also work against other cancers.
In 1992, the Kimmel Cancer Center earned a new type of funding offered by the National Cancer Institute to speed the translation of laboratory research to new diagnostics and therapeutics for cancer. The program was called SPORE, for Specialized Projects of Research Excellence. The Kimmel Cancer Center was the only NCI-designated cancer center to earn multiple SPOREs.
In 1992, the Kimmel Cancer Center earned a new type of funding offered by the National Cancer Institute to speed the translation of laboratory research to new diagnostics and therapeutics for cancer.

One SPORE helped Baylin and team advance epigenetic discoveries. They began using methylation levels as an early indicator of a developing cancer and to predict whether a cancer would respond to specific cancer drugs, and if it was likely to come back after treatment. Kimmel Cancer Center epigenetics researcher and surgeon Malcolm Brock, M.D., used epigenetic markers as a guide during lung and esophageal cancer surgeries to help him determine if all of the cancer was removed. He dubbed it molecular staging. Although there was no visible evidence of cancer, Brock and others were using methylation as an epigenetic trail of evidence to reveal cancer cells hiding in tissue, such as lymph nodes.
Working with Herman, Brock revealed that the evidence of small cell lung cancer’s inevitable return was in the methylation patterns of four genes. Depending upon the combination of genes abnormally methylated, the risk of a cancer returning was 2% to 25%. The overmethylation of two genes — p16 and H-cadherin — proved to offer the worst scenario, foreshadowing a swift return of the cancer.
In 2004, their body of epigenetic research was recognized by the NCI as the most outstanding in the SPORE program.
Similar work soon expanded to prostate, head and neck, breast and other cancers.
Breast Cancer Test

Breast cancer researcher Saraswati Sukumar, Ph.D., modified a test called MSP to detect breast cancer. The test was originally developed by Baylin and Herman in 1996 and used in the Cologuard test to detect abnormally methylated genes in stool samples. The Liquid Biopsy for Breast Cancer Methylation test measures DNA methylation in 10 tumor-specific genes from fluid aspirates of the breast lesion and sometimes an enlarged lymph node. The test has three cartridges that hold chemicals to detect methylated DNA from fluid obtained from the patient’s breast growth or lymph nodes, and includes a computer pre-loaded with software to analyze the data and return results within five hours. The test can detect new breast cancers and help detect breast cancer recurrence.
“Our goal was to develop an assay that would be sophisticated yet simple to perform worldwide and could be used at the point of care to provide same-day feedback to clinicians and patients,” Sukumar says.
Clinical Studies
Nilofer Azad, M.D., director of the Center’s Colorectal Cancer Research Center of Excellence and the Developmental Therapeutics Program, led clinical studies of epigenetic-targeted therapies in colon cancer. She worked to develop epigenetic biomarkers that predicted sensitivity to specific chemotherapy drugs.
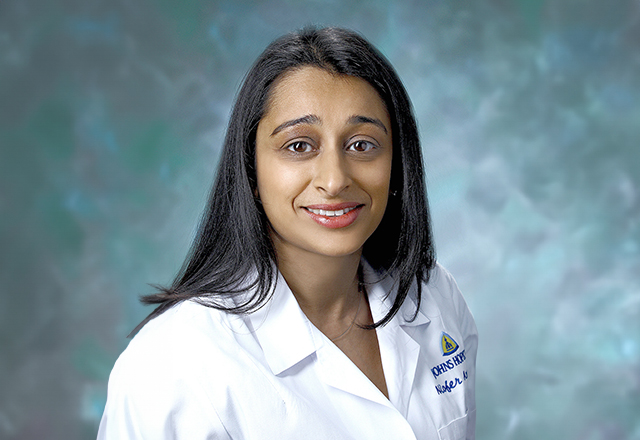
She used the MSP test developed by Baylin and Herman to identify a specific epigenetic biomarker that indicates cancers that should be susceptible to a class of anticancer drugs called taxanes, which were once thought ineffective in colon cancer. With drug treatments for colon cancer limited, Azad believes an individualized epigenetic approach could significantly expand the options for patients.
“Many drugs have been tested and looked inactive when they are given broadly to large groups of patients, but we are finding that there are subsets of patients who may benefit, and we can use epigenetic biomarkers to identify these patients,” says Azad. “Drug treatments are limited for colorectal cancer patients, and this is one approach that could help us significantly expand options for patients.”
Dream Teams
In 2008, the Entertainment Industry Foundation and Major League Baseball formed Stand Up To Cancer (SU2C) to mobilize the public to donate money for cancer research and to motivate the scientific community to collaborate on promising areas of research that could quickly be moved to clinical trials. “Dream teams” made up of clinicians and scientists from across the country — the best in their fields — were selected after rigorous review by another panel of esteemed cancer experts and directed to take on specific cancer research projects. When the epigenetics dream team was announced, Baylin was selected as co-leader.
DNA Packaging
His research of the role of DNA methylation in cancer had led him to a molecular co-conspirator. He observed that it wasn’t just DNA methylation that affected gene expression but also the way DNA was packaged in a cell. It reflected Nobel Prize-winning work on how DNA is wrapped in a structure, called the nucleosome, an area of research also extensively studied by Jones.
The nucleus is a structure so tiny that more than 50,000 of them can fit on the head of a pin. If the DNA contained within one cell was extracted and stretched out end to end, it would extend 6 feet, yet all of that molecular material is compacted and packed inside the nucleus of a human cell. Chromatin, a complex combination of proteins, mainly histones, which defines the nuclesosome, is responsible for compressing the DNA to fit inside a cell.
This packaging also plays a role in gene expression and the copying of DNA as cells divide. A loose chromatin results in normal gene expression, but add methylation to the mix, and this compacts the position of nucleosomes on DNA and silences gene expression. Baylin and team found this tightened chromatin could keep genes, including tumor suppressor genes, in a constant state of non-expression. It also caused cancer cells to behave in a primitive, embryonic-like manner. Unlike normal embryonic cells, which receive and respond to signals that tell them to stop making new cells, epigenetically altered cancer cells maintain their ability to replicate, renew and divide.
Scientists do not know what prompts the cancer-promoting changes in chromatin structure. They suspect it may be a repair mechanism engaged in response to cell injury, such as chronic inflammation. Baylin and colleagues reported that these dynamics can initiate and maintain the abnormal DNA methylation, associated tumor suppressor gene silencing, and the malignant properties of established cancer cells. In the laboratory, when he and his team combined a demethylating drug with a histone-blocking drug (HDAC inhibitor) in human cancer cell lines, the chromatin structure loosened, and some gene expression was restored. Blocking inflammation-induced dynamics can have the same result, says Baylin. These discovery findings were the focus of the first SU2C Epigenetic Dream Team patient studies.
Combinations
The first clinical study of the combined demethylating agent and histone-blocking HDAC inhibitors was in patients with advanced lung, breast and colon cancers. The drugs were not given at the highest dose that patients could tolerate, as is usually the case in early studies of anticancer drugs. Rather, low doses were given. The goal was to kill the cancer cells by reprogramming their DNA, instead of obliterating them with chemotherapy agents. In essence, the researchers were using the drugs to convert cancer cells back to normal cells.
At high doses, the drug killed cancer cells, but at lower doses over time, it reprogramed cancer cells to behave like normal cells, a much less toxic and more permanent cancer fix. It was a radical departure from the standard approach of blasting cancer cells with as much poison as possible, but there was significant laboratory evidence to show that it could work.
The responses, although small in number, were unprecedented. Patients with resistant, lethal lung cancer that had spread to other organs and was resistant to other treatments were seeing their tumors melt away. In a few other patients, tumors stopped growing. The cancers didn’t go away, but they seemed to be dormant.
Still, most patients treated did not respond, and responses in patients with breast cancer and patients with colon cancer were not nearly as dramatic as those seen in the small group of patients with lung cancer. This did not surprise or deter Baylin and team. Earlier work by him, Herman and Brock showed that specific epigenetic biomarkers provided a signature that could differentiate patients who were likely to respond from those who would not.
This trial was open to all patients with resistant cancers, and with no analysis for the epigenetic signature of their tumors, the expectation was that a small subset of patients would see results. The analysis would come later with Baylin, basic scientist Cynthia Zahnow, Ph.D., and cancer surgeon and former faculty member Nita Ahuja, M.D., taking cells back to the laboratory for gene expression analyses.
Reigniting Responses
With funding from SU2C, Baylin and team had the opportunity to follow up on patients who were taken off the trial because their cancers continued to grow despite treatment with the experimental epigenetic therapy. These patients had end-stage cancers that had spread and were unresponsive to three different attempts at chemotherapy, so they expected most had passed away. However, when the team went back and reviewed the records of these patients, they learned that many of the patients with lung cancer were still alive because their cancers had suddenly begun to respond to a wide variety of anticancer drugs.
Patients whose tumors seemed to progress while they were on the experimental therapy — some who had only received two or three treatments — were alive and doing well. Cancers that had continued to grow and spread despite every effort were suddenly transformed. They pored over every scan, piece of clinical paperwork and biopsy report available.
“There could only be two explanations,” says Baylin. “Either the epigenetic therapy sensitized the cancers to subsequent treatment with standard drugs, or their improvement was a direct response to the epigenetic therapy.”
Priming Effect
The team needed to complete further studies in the laboratory to solve the mystery.
These new epigenetic-targeted therapies do not work like the old cell-killing cytotoxic chemotherapies that do not discriminate between normal cells and cancer cells. Instead, Baylin says they worked slowly over time as they made repairs and returned genes to normal function. Baylin, Herman, Brock, Ahuja and Zahnow also found that the epigenetic drugs had a priming effect on the tumor and made formerly resistant cancer cells begin responding again to treatment with anticancer drugs.
Immune Evasion

As they began to study the cell lines in the laboratory, they found that the epigenetic drugs had the capability to impact almost every type of cell mechanism, including cell division,
cell repair, and cell cycle and death. Of particular interest to the researchers was the treatment’s effect on genes related to immune response.
Immune cells are on patrol at all times in the human body, differentiating between foreign invaders and normal cells. Cancer cells are derived from normal cells, so they can fly beneath the radar of the immune system. However, as the science of cancer immunology has advanced, researchers are finding that there is more to the cancer cell’s ability to evade the immune system than its similarities to normal cells. Cancer cells use epigenetic controls to corrupt immune responses to cancer cells. By hijacking the mechanisms that allow the immune system to differentiate an invading virus cell from a body’s own cells, it causes the immune system to tolerate cancer.
In their laboratory analyses of gene expression in cell lines derived from patients in the epigenetic treatment studies, one immune target jumped out at them. This target was a gene called PD-L1.
Epigenetic treatment turns on a number of silenced genes. Some of them encode molecules in the immune system that turn on immune responses and some that turn them off and lead to immune evasion. Immune-inhibiting genes turned on by epigenetic therapy include PD-1, part of the intricate checkpoint system hardwired into the immune system, and its partner PD-L1.

Normal human cells need the ability to communicate with immune cells that they are the good guys and should be left alone. Unfortunately, cancer cells exploit the same process to avoid an immune attack.
Baylin and Zahnow sought out the help of Cancer Center immunology expert Drew Pardoll, M.D., Ph.D. In some patients in the study, the PD-L1 gene was already active, and laboratory studies indicated that its expression by lung cancer cells might be enhanced by epigenetic therapy. Pardoll believed that using a drug to block PD-L1 or PD-1 in conjunction with epigenetic therapy could alter the balance of immune effects of the treatment toward an activated immune response right within the tumor.
Pardoll recruited the help of other Kimmel Cancer Center colleagues, including cancer immunology expert Suzanne Topalian, M.D., and lung cancer expert Julie Brahmer, M.D.
It has been well established that cancer has an immune evasion signal. To survive, cancer cells need to at least partially adapt to their environment. They send out a “don’t look at me” signal to immune cells. Treated with epigenetic drugs, however, the ability to evade the immune system is broken and cancer cells send new signals — on one hand, they beckon the immune cells to come and get them, and on the other, they shield against immune attack by expressing PD-L1.
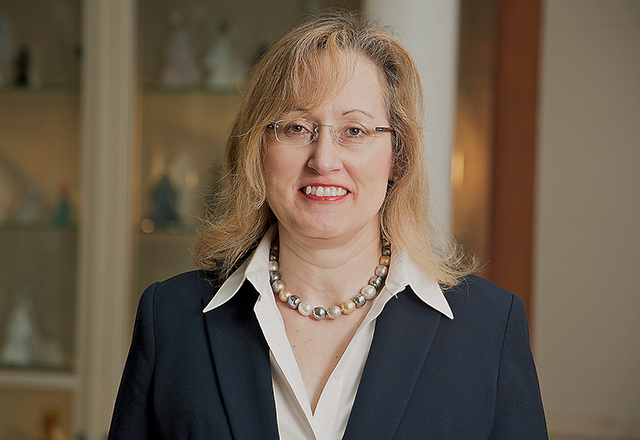
Back to the Lab
Baylin, Zahnow, Ahuja and colleague John Wrangle, M.D., went back to the laboratory to decipher the immune evasion signature for lung, breast, colon and ovarian cancers. To do this they looked at all of the genes that get turned on in cancer cells with demethylating drugs. Lots of genes, they found, get reactivated, but about 20% of them are related to immune regulation. Their findings revealed that a significant part of what the epigenome does is regulate the immune system.
Their research revealed a set of genes that are epigenetically programmed to evade detection by the immune system. Using a drug to reverse this programming may force the cancer cells out of hiding and make them more vulnerable to treatment, or even better, allow the immune system to see the cancer and kill it.
SU2C support included a study of a combined therapy of a demethylating agent, a histone-blocking HDAC inhibitor and anti-PD-1 treatment.
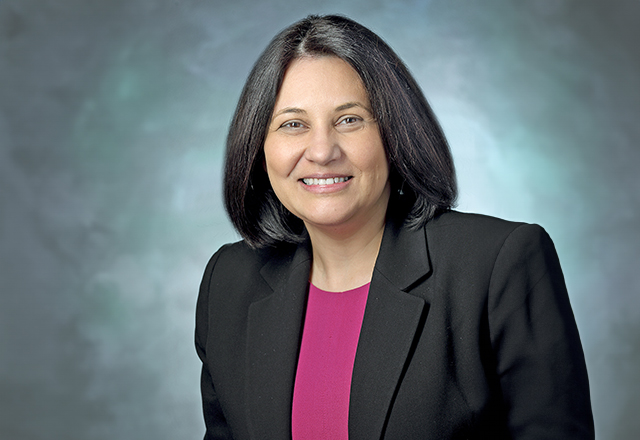
There were two components to the trial, one aimed at verifying the immune responses and the other at further testing the epigenetic priming effect — the ability of epigenetic therapy to sensitize cancers to subsequent chemotherapy. At the same time, a number of trials were launched at the Kimmel Cancer Center and elsewhere studying a wide variety of epigenetic drug combinations and single agents.
Kimmel Cancer Center experts accumulated 70 cell lines from breast, colon, ovary and lung cancers and patient biopsies that they were comparing to the cell lines. Gene expression data, methylation data, proteomics data — anything that could be measured in a cancer cell was being analyzed.
There is some evidence that demethylating agents have a stronger effect on the epithelial cells where cancers most often originate. The histone-blocking HDAC inhibitors appear to influence the immune cells and microenvironment.
Other Kimmel Cancer Center experts also believed that epigenetic therapy might be more powerful in combination with immunotherapy.
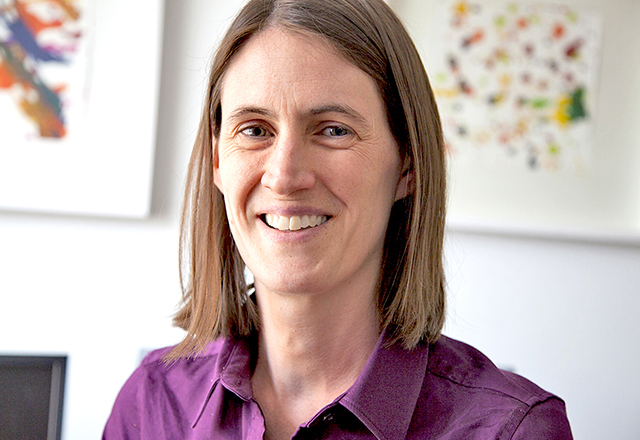
Epigenetic alterations are common in breast cancer, so breast cancer experts Vered Stearns, M.D., Roisin Connolly, M.D., and Evanthia Roussos Torres, M.D., Ph.D., collaborated with leading cancer immunology expert Elizabeth Jaffee, M.D., deputy director of the Kimmel Cancer Center, to develop a study of combined epigenetic/immune therapy for breast cancer. In their study, they gave an epigenetic drug called an HDAC inhibitor two weeks before treatment with an immunotherapy that released restraints on immune cells in an effort to prime the immune response to the cancer. They continue to study tumor samples and blood samples from patients to identify biomarkers that help identify those most likely to benefit from the combination therapy.
“There was still so much we needed to learn,” says Zahnow. “What is the best way to give the drugs? Should they be given simultaneously or consecutively? What are all of the targets the drugs hit?”
Epigenetics and Genetics
Cancer genetic and epigenetic research has advanced dramatically at the Kimmel Cancer Center, with the leading experts in both disciplines working together. The interplay between genetics and epigenetics was revealed because of Kimmel Cancer Center excellence in both fields.

“It’s interrelated,” says Vasan Yegnasubramanian, M.D., Ph.D., who runs the Kimmel Cancer Center Next Generation Gene Sequencing laboratory. “Many epigenetic problems may have their basis in genetic abnormalities. The genes that get mutated in cancer are often genes that control DNA packaging.”
A prime example of a genetic mutation having epigenetic consequences is the brain cancer gene called IDH1, identified by Ludwig Center cancer genetics researcher Nickolas Papadopoulos, Ph.D., and team in 2008. IDH1 produces an enzyme that regulates cell metabolism, but a mutation in the gene results in increased production of a metabolite that can affect DNA methylation. IDH1 mutations are very simple genetic changes, but they cause a cascading effect of alterations to the epigenetic landscape that ultimately become a major driving force behind the cancer.

Investigators believe there are many more examples of the genetic/epigenetic collaboration in cancer. Although it is impossible to fix a mutated gene, the epigenetic changes can be targeted and disrupted with drugs.
In a study of prostate cancers from men who died of the disease, Yegnasubramanian found increased methylation in genes not methylated in normal tissue. In each patient studied, this pattern of hypermethylation was consistently maintained across all of the metastatic prostate tumors and occurred near genes in cancer-related pathways that control development and differentiation.
If we looked at all of the genes silenced epigenetically in cancer and could turn them all back on, no cancer cell could withstand it."
NELSON![]()
“We need to do more research, but it looks like the areas that have increased methylation are being selected by the cancer cell to keep its advantage,” says Yegnasubramanian. “We know these were resistant cancers because we obtained the tumor samples from men who died of prostate cancer. Perhaps if these methylation alterations could have been reversed, the cancer cells might become sensitized to treatments.”
The opportunity to offset the collateral damage to epigenetic functions caused by broken genes is one of the newest and most promising iterations of epigenetic research, and one that is rapidly revealing new targets for treatment. Driving this progress is new technology that allows investigators to catalog epigenetic changes and align them back to the genome.
“There are striking differences in how DNA is organized in the cancer cell and how it is organized in the normal cell,” says Yegnasubramanian. “Now we have the technology to go in and look at this at the molecular level.”
This ability has become critically important with growing evidence that some mutated tumor suppressor genes establish cancers through many subsequent epigenetic alterations.
“Although the mutation is the initiating event, it is the epigenetic alterations that are involved in driving the cancer, and unlike mutations, the epigenetic changes can be targeted and halted with drugs,” says Yegnasubramanian.
A Cancer Reset
In this era of personalized cancer medicine, many experts believe that epigenetics could be a master control of sorts, so intrinsic to the initiation and spread of cancer that it could potentially provide opportunities to globally reset cancer cells. The panel of epigenetic alterations that drive a particular cancer may vary, but if they can be identified in individual patients, then maybe we have found the Achilles’ heel of cancer.
Still, most experts agree that science has only scraped the surface when it comes to epigenetics. The understanding of the full power of epigenetic mechanisms to read, write, erase and move genetic code is just beginning to be understood, but already we have promising treatments.
“If we looked at all of the genes silenced epigenetically in cancer and could turn them all back on, no cancer cell could withstand it,” says Nelson. “We can do that in the laboratory, and now we are learning how to do it safely and effectively in humans. We have tremendous opportunity and unparalleled ingenuity. All we need to do is connect the dots.”