Illustration by Andrew Colin Beck | Photos by Chris Myers
Half a century is an eternity in modern medicine.
Back in the 1970s, patients with diabetes didn’t have metformin. Heart patients weren’t taking ACE inhibitors or beta blockers. Compared with today’s meticulously calibrated cocktails, chemotherapy regimens were brutal and primitive.
Diabetes, heart disease and cancer still rank as killers, of course, but they were much deadlier 50 years ago. Similar success stories can be told in countless other clinical specialties, thanks to drugs, devices and other therapeutics that have saved or extended millions of lives.
Johns Hopkins neurosurgeon Nicholas Theodore wishes he had a story like that to tell his patients. Alas, no significant new drugs or treatments have arrived on the spinal cord injury front over those past five decades.
Nearly 20,000 Americans suffer these debilitating injuries every year, mostly due to auto accidents, falls and violence. Mortality rates are quite high through the first year after an accident, especially due to cardiovascular complications. Those who survive — and there are about 300,000 living with old injuries in any given year — face elevated risks for potentially fatal complications associated with blood clots, pneumonia and urinary infections. Life expectancy among these survivors is markedly lower than that of the general population.
This unfortunate state of affairs is what motivated Theodore to leave the prestigious Barrow Neurological Institute in Phoenix, Arizona, and come to Johns Hopkins in 2016. The move promised better access to world-class multidisciplinary expertise, especially in biomedical engineering. That’s the cutting edge where Theodore hopes to find an alternative to what he calls “the same rudimentary things that we’ve been doing in these cases for 50 years.”
In the hours or days after injury, a neurosurgeon will open up the patient and “decompress” the spinal cord, clearing out stray pieces of bone and pockets of hematoma so as to stabilize the spine. Physicians monitor blood pressure very carefully in the post-surgical period, tinkering frequently to make sure nothing is awry with perfusion and blood flow.
The weeks after surgery are fraught with risk, especially as inflammation arises and scar tissue forms. The resulting complications can cause worsening neurological injury. Outside of trying to manage these crises, however, the treatment regimen ahead is mostly a matter of helping patients adjust to their new realities.
“From the moment of impact, lives are changed forever,” Theodore says. “These are devastating injuries. You can’t walk. You lose the use of limbs. You’re paraplegic. You’re quadriplegic. And we don’t really have any therapeutics to offer, nothing that might start to reverse the damage and let patients regain some functioning.”
But Theodore has high hopes that his latest project can change this state of affairs. Late in 2020, a team he heads in concert with Johns Hopkins biomedical engineer Amir Manbachi won a five-year, $13.48 million grant from the Defense Advanced Research Projects Agency (DARPA). The scope of the project is quite ambitious — “an engineering and medical tour de force,” in Theodore’s words, that has the potential to deliver therapeutic progress for spinal injury patients at long last.
Plugging the Dike
The lack of progress in spinal cord therapeutics does not reflect a lack of effort. Scores of drugs offering glimmers of hope have entered clinical trials in recent decades. Not a single one has passed regulatory muster. In the early 2000s, stem cell therapies seemed tantalizingly close to arriving at the bedside. Paralyzed actor Christopher Reeve became a cause celebre in those days, with much attention paid to his fervent belief that there was “a cure coming” for him along the stem cell path. He died in 2004. Research into stem cell therapies continues today, minus that buzz about imminent breakthroughs.
Today’s next-big-thing buzz belongs to treatment with electric stimulation. Physicians have known for some time now that by aiming low-level electric impulses at specific paralyzed muscles, they can spark contractions. Treatment along these lines helps some patients regain muscle mass and perhaps expand their range of motion. Most insurance companies still classify electric stimulation as experimental, however.
In recent years, researchers have started playing with the concept of sending electrical impulses along the spinal cord itself, rather than simply targeting a muscle. In a few limited but well-publicized cases, patients have regained lost mobility. The theory is that such stimulation boosts the power of signals from the brain telling this or that muscle to move. The work remains at an early stage, however, and Theodore has seen exciting concepts come and go before.
“I’ve been involved in research in this field for 25 years — drug trials, experimental therapeutics, all of it,” he says. “I don’t mean to be a nihilist. But the fact is, nothing has worked.”
Theodore’s journey to the new DARPA project began with the obvious question: Why have so many therapy candidates failed? He calls on an old saw of a folktale to put this in perspective — the one about a Dutch boy who puts his finger in a hole in a dike to prevent a flood. Spinal cord research tends to follow that Dutch-boy model: See a little hole, plug a little hole. What gets little attention, he says, is how this or that little hole fits into the whole of a flood wall.
“We see very complicated cascades of events on the cellular level in these injuries,” Theodore says. “The cascade that happens in the immediate aftermath is just the beginning. After that come secondary injuries — loss of blood flow, loss of oxygen, inflammation and scar tissue forming.”
Each of those can spark another cellular cascade, and these too involve dizzying complexities. Along the way, the body loses its knack for autoregulation. No longer can it manage the process of figuring out how much blood should flow through this artery or when this or that electrical impulse should fire.
Theodore circles back to his Dutch boy: “If you’ve got 50 holes in a dike and you stick your finger in one of them, you’re still going to drown,” he says.
Seeking fresh eyes, Theodore paid a visit in 2016 to the Johns Hopkins Whiting School of Engineering and put a question to Manbachi: How can we give spinal cord researchers a clearer view of that full flood wall and its interrelated holes?
The first thing Manbachi did was turn Theodore’s question into a teaching moment, asking the neurosurgeon to present his question to engineering students. The challenge was akin to a product-development visioning exercise: What sort of device or devices might help researchers move beyond that Dutch-boy way of thinking?
Undergraduate Ana Ainechi soon formed and led an engineering capstone design team. Working with guidance from Manbachi and Theodore, that team played a key part in the work that followed. Four years later, Ainechi is still working in Manbachi’s lab on the questions raised in Theodore’s presentation.
The Ultrasound Band-Aid
Manbachi is no stranger to working with neurosurgeons. His lab specializes in developing cutting-edge acoustic probes and ultrasound transducers that work in neuroscience settings. The solution he and his students came up with in this case involves a pair of devices with names familiar enough to sound humdrum. One is ultrasound; the other is a catheter. But there is nothing humdrum about either device, or about the way the two are designed to work together on a machine learning level.
Ultrasound made its first splash as a clinical tool during the 1970s, once the invention of rudimentary scanners gave physicians a way to visualize and process the information available through high-frequency sound waves. Best known as a way to view a fetus in utero, ultrasound quickly became an everyday tool across scores of specialties. Think echocardiograms in cardiology or bone sonometry in orthopaedics. Other examples abound.
Like most electronic devices, ultrasound machines have grown smaller and gotten more powerful over time. The challenge Manbachi’s lab is tackling involves pushing those trends into uncharted territory. He’s out to build the smallest ultrasound machine ever made, something about the size of a Band-Aid. He wants to make it biocompatible, too, as the idea is to stick that Band-Aid on the spinal cord during surgery. No one has ever made such a device before.
“This is as pioneering as it gets, very ambitious,” Manbachi says. “But if we’re able to do it, we’ll be able to see, in great detail, what’s happening in the tiniest microvasculature of the spinal cord.”
Then there is the catheter. Designed to float in a patient’s spinal fluid, it will be “impregnated” with highly sophisticated electronics and provide constant reads on spinal fluid pressure, temperature and other biomarkers. Manbachi’s team is hoping it can measure oxygenation as well; time will tell on that front.
If the technology works, this one-two punch will give physicians access to a wealth of previously hidden biological details about what’s happening in the microenvironments around a spinal cord injury. They will gain a new understanding of the blood flow disruptions that occur both in the initial injury and during those secondary cellular cascades, which often lead to increased inflammation, excruciating pain and worsening paralysis.
To illustrate the extent of the transformation on the horizon, Theodore contrasts the current state of blood pressure monitoring in spinal cord surgeries with those employed in brain surgeries.
“We’re far more sophisticated in the brain right now,” he says. In neurotrauma, surgeons drill a hole in the head and insert a tube in the fluid-filled space of the brain, gauging intracranial pressure.
“But the anatomy of the spinal cord is different — its diameter is about the size of your pinky finger,” he continues. “I can’t stick something into the spinal cord without causing injury, and that means I can’t measure pressure the way I can in the brain.” That leaves surgeons making decisions about whether to raise a patient’s blood pressure based on readings from a standard arm cuff.
“What we’re talking about here,” Theodore continues, “is the ability to see what’s happening with pressure not in the whole body, but in blood vessels that are smaller than a strand of human hair. That’s why this is exciting. The way things stand now, it’s like the surgeon is up on a tightrope with a blindfold. We’re flying by the seat of our pants, no real information to work with. With this, the blindfold would come off. It would create tremendous opportunities for progress.”
Among the possibilities is the elusive goal that brought Theodore to Hopkins — therapeutics. Both devices have roles to play on this front. Researchers in several specialties have been experimenting in recent decades with the use of low-intensity pulsed ultrasound to help stimulate blood flow — and, often, healing — around various types of wounds. On a more cutting-edge front, researchers have begun looking at ultrasound as a way to drive piezoelectric current along the spine, perhaps putting the DARPA project in the thick of work on that aforementioned next-big-thing candidate: electric stimulation therapy.
The catheter, too, will have potential as a therapeutic tool. Its array of electronics is being designed by a team of specialists at the Johns Hopkins University Applied Physics Laboratory headed by Monique Beaudoin, who manages APL’s Neurological Health and Human Performance program. The idea is to equip the device with machine learning skills so that it processes incoming data from spinal microenvironments and then does a much better job of deciding on drug dosages or identifying ideal times to drain spinal fluid.
The two devices will be in constant wireless communication. Manbachi envisions a day when patients will have apps on their phones that help them understand why they may not be feeling 100 percent — and what they can do about it.
Theodore, too, finds himself daydreaming about where the DARPA project might lead. But he’s also keeping his focus on essential first steps toward genuine clinical progress.
“Just having these two devices communicating with each other and learning in real time about what’s going on for an individual patient, that will be big to start off with,” he says. “It will help us answer a lot of questions. What can we do to improve perfusion? How can we save spinal cord tissue that’s at risk? We can change outcomes for patients if we do that.”
As an example, he points to a common turn of events in spinal injury cases. An initial injury leaves a patient unable to use a hand, but still able to move an arm. But then the arm, too, becomes useless, due to one or another of those secondary cellular cascades.
“If we can change just those outcomes, saving something like that arm mobility, it will make a big difference for patients,” Theodore says. “There’s a lot of lofty goals here. We’d all love to find a way to ‘cure’ spinal cord injury. But our first steps toward good therapeutics will be baby steps, and we need to remember that those are going to help patients, too.”
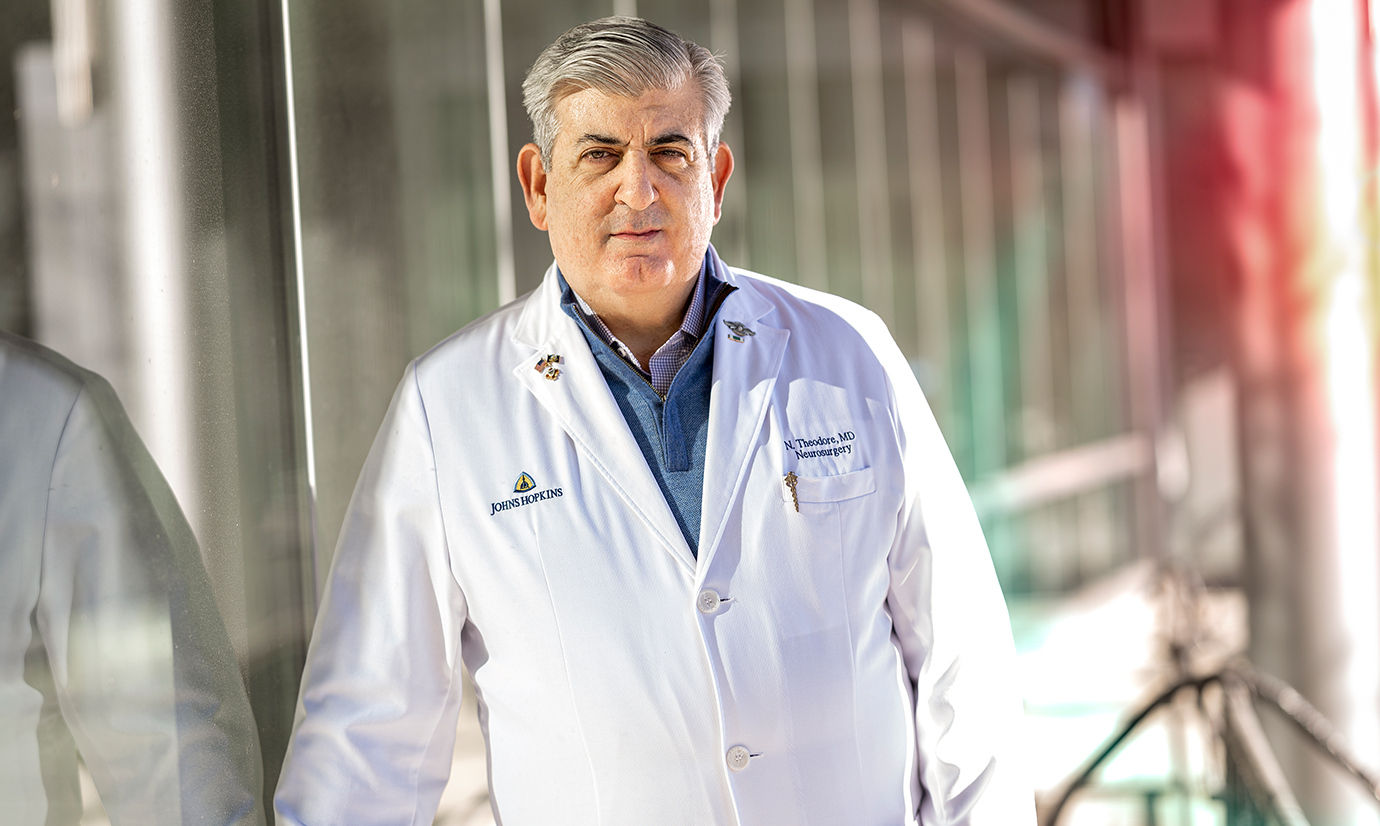
Think Like a Startup
An agency of the U.S. Department of Defense, DARPA is best known for its support in the 1960s of a fledgling data platform called ARPANET, a key precursor of the modern internet. The agency prides itself on backing high-risk, high-reward projects. That, in turn, means DARPA operates with a higher tolerance for failure than most other research funders — the agency isn’t afraid to place bets on longshots.
That makes DARPA projects different. The way Theodore sees it, the agency wants research teams to act “more like startup companies” and less like traditional academic labs. Consider the case at hand. If Manbachi and Theodore pursued this one-two punch of ultrasound and catheter through, say, the National Institutes of Health, they would end up running, one by one, through a sequence of different grants.
One might demonstrate the feasibility of ultrasound miniaturization. Another might look to develop the machine learning catheter. Eventually, they’d seek a grant to test their ultrasound Band-Aid on a pig. If that worked, the application process would start again, this time in search of yet another grant to test the device in humans.
“It would probably take decades,” Manbachi says.
DARPA wants to see that run happen in five short years. The 2025 end point here is two working devices that have passed muster in an animal model and are ready to be tested in human beings.
“In traditional research, we understand what that kind of end result might be,” Theodore says, “but we’re never really planning that far ahead. We’re just dealing with one step at any one time.” He suspects that the number of simultaneously moving parts in this DARPA project would come as quite a shock to researchers in academic labs accustomed to more traditional approaches.
“We don’t even have a device yet, but we’re already talking with the Food and Drug Administration about biocompatibility,” Theodore says. “We’re working on commercialization questions, too. How does this get patented? How does it get manufactured? You might be the smartest engineer in the world, but if what you make doesn’t have a profitable and protected pathway that lets a company get it into the hands of surgeons, it’s not going to be successful.”
Another DARPA difference: The grant is organized around a sequential series of milestones along that five-year timeline. Manbachi and Theodore will need to hit each new milestone in lily-pad fashion in order to make it all the way through that full funding pool of $13.48 million.
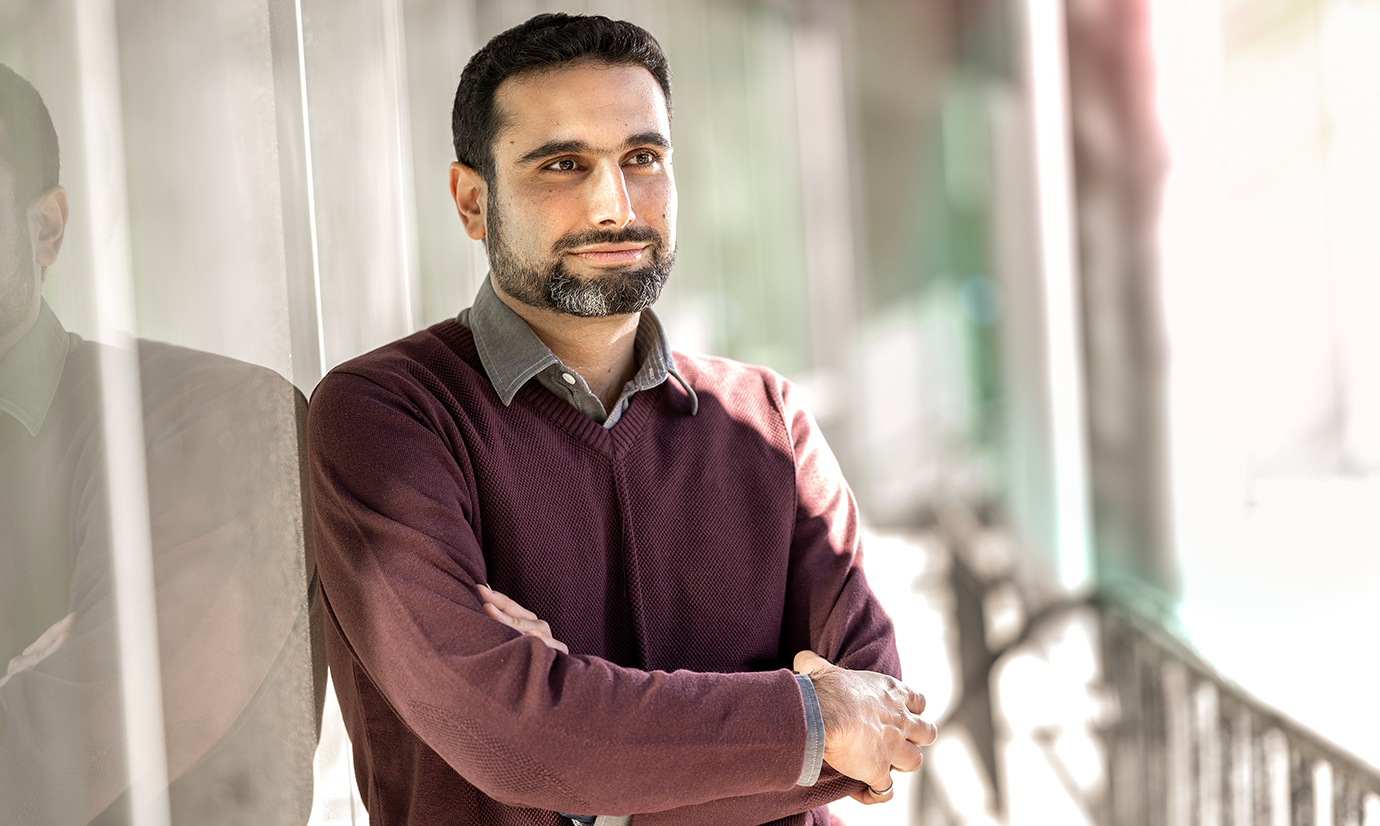
The Data Difference
If things go according to plan, that five-year finish line may end up becoming the start of something much bigger. Manbachi likes the way a colleague at DARPA describes the project at hand as a “system of systems,” all working seamlessly together to gather and respond to biological data gleaned at an unprecedented level of detail. Inventing the parts that make up that system will necessitate innovation at eye-popping, attention-getting levels — from building the world’s smallest ever ultrasound machine and implanting it inside the human body to transforming a modest little catheter into a high-powered, self-directed, machine learning diagnostician.
But the most significant outcome of the DARPA project may arrive in less flashy fashion, through the simple accumulation of these detailed data over time, across one and then dozens and, eventually, thousands of patients.
“Remember,” Manbachi says, “these systems are going to get smarter and smarter as they work together.”
As an example, Manbachi and Theodore point to a potential military application. All of DARPA’s projects are tied to the mission of the Department of Defense, and this one is no different, as spinal cord injuries are common on the battlefield. Theodore brings relevant experience to that aspect of the project, having served for more than 10 years as a neurosurgeon in the U.S. Navy, treating soldiers and sailors with spinal cord injuries.
At the moment, military physicians have no way of making timely, in-theater decisions about which injuries are serious enough to merit transport from an in-theater facility to a full-fledged military hospital that is often thousands of miles away.
“Think this through, and you’ll see why the machine learning aspect is vital,” Manbachi says. “You’ll be able to store all these data, and then compare things after 100, 500, 1,000 patients. You’ll finally have the information you need to identify the high-risk cases and choose the right treatment strategies.”
Similar advances will unfold in civilian neurosurgery units, where researchers will put their reams of data to use in evaluating promising proposed treatments, whether that’s electrical stimulation or some future next-big-thing possibility. Theodore also finds himself wondering what physicians in other specialties are going to make of this “system of systems” once it’s up and running.
“I think this is going to open up the potential for therapies that we’ve not even thought of right now,” he says. “We’re working here at the intersection between engineering and medicine, and there is a lot of history in this area of devices being developed for one purpose but soon filling others.”
He cites the DaVinci surgical robot as an example. It was developed with heart surgery in mind and later adapted to work in prostate surgery and gynecologic surgery.
“The ability to make these measurements in a microenvironment with implantable devices might end up being used in the brain or on the liver — who knows where else?” he says. “We’re working at the tip of a very big iceberg here. I really believe that this has the potential to transform clinical medicine.”